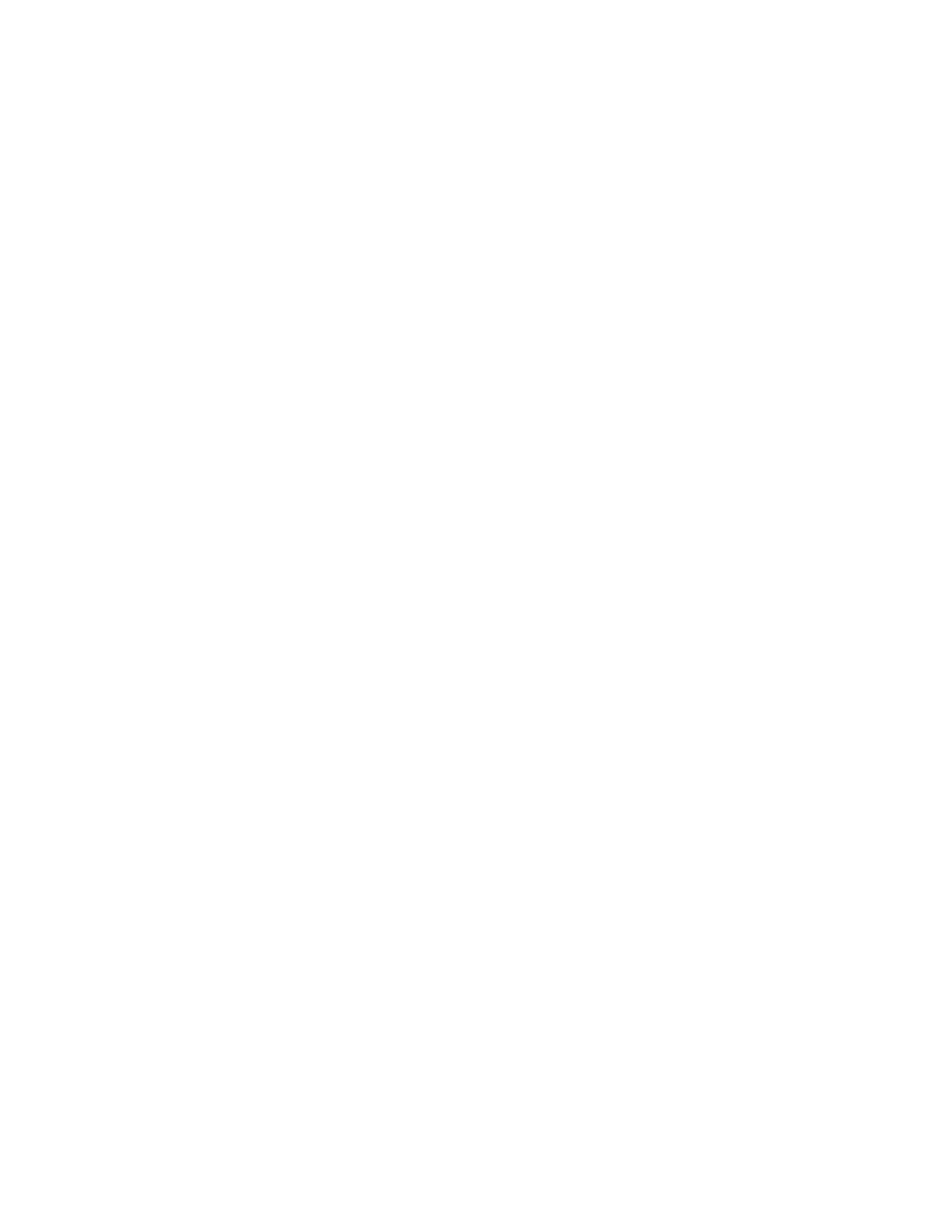
conductivity at the reference electrode (solvent) and the analyt-
ical electrode (solvent ⫹carrier ⫹reaction products).
2) Electron capture detector—The electron capture detector
(ECD) usually is used for the analysis of compounds that have
high electron affinities, such as chlorinated pesticides, drugs, and
their metabolites. This detector is somewhat selective in its
response, being highly sensitive toward molecules containing
electronegative groups: halogens, peroxides, quinones, and ni-
trogen groups. It is insensitive toward functional groups, such as
amines, alcohols, and hydrocarbons.
The detector is operated by passing the effluent from the gas
chromatographic column over a radioactive beta particle emitter,
usually nickel-63 or tritium adsorbed on platinum or titanium
foil. An electron from the emitter ionizes the carrier gas, pref-
erably nitrogen, and produces a burst of electrons. About 100
secondary electrons are produced for each initial beta particle.
After further collisions, the energy of these electrons is reduced
to the thermal level and they can be captured by electrophilic
sample molecules.
The electron population in the ECD cell is collected periodi-
cally by applying a short voltage pulse to the cell electrodes and
the resulting current is compared with a reference current. The
pulse interval is adjusted automatically to keep the cell current
constant, even when some of the electrons are being captured by
the sample. The change in the pulse rate when a sample enters
the ECD is then related to the sample concentration. The ECD
offers linearity in the range of 10
4
and subpicogram detection
limits for compounds with high electron affinities.
3) Flame ionization detector—The flame ionization detector
(FID) is widely used because of its high sensitivity to organic
carbon-containing compounds. The detector consists of a small
hydrogen/air diffusion flame burning at the end of a jet. When
organic compounds enter the flame from the column, electrically
charged intermediates are formed. These are collected by apply-
ing a voltage across the flame. The resulting current is amplified
by an electrometer and measured. The response of the detector is
directly proportional to the total mass entering the detector per
unit time and is independent of the concentration in the carrier gas.
The FID is perhaps the most widely used detector for gas
chromatography because of several advantages: (a) it responds
to virtually all organic carbon-containing compounds with high
sensitivity (approximately 10
⫺13
g/mL); (b) it does not respond
to common carrier gas impurities such as water and carbon
dioxide; (c) it has a large linear response range (approximately
10
7
) and excellent baseline stability; (d) it is relatively insensi-
tive to small column flow-rate changes during temperature pro-
gramming; (e) it is highly reliable, rugged, and easy to use; and
(f) it has low detector dead volume effects and fast response. Its
limitations include: (a) it gives little or no response to noncom-
bustible gases and all noble gases; and (b) it is a destructive
detector that changes the physical and chemical properties of the
sample irreversibly.
4) Photoionization detector—Photoionization occurs when a
molecular species absorbs a photon of light energy and dissoci-
ates into a parent ion and an electron. The photoionization
detector (PID) detects organic and some inorganic species in the
effluent of a gas chromatograph with detection limits as low as
the picogram range. The PID is equipped with a sealed ultravi-
olet light source that emits photons which pass through an
optically transparent window (made of LiF, MgF
2
, NaF, or
sapphire) into an ionization chamber where photons are absorbed
by the eluted species. Compounds having ionization potential
less than the UV source energy are ionized. A positively biased
high-voltage electrode accelerates the resulting ions to a collect-
ing electrode and the resulting current is measured by an elec-
trometer. This current is proportional to the concentration.
The PID has high sensitivity, low noise (approximately 10
⫺14
A), and excellent linearity (10
7
), is nondestructive, and can be
used in series with a second detector for more selective detec-
tion. The PID can be operated as a universal detector or a
selective detector by simply changing the photon energy of the
ionization source. Tables of ionization potentials are used to
select the appropriate UV source for a given measurement.
5) Mass spectrometer—The mass spectrometer (MS) has the
ability to detect a wide variety of compounds, coupled with a
capacity to deduce compound structures from fragmentation
patterns. Among the different types of mass spectrometers, the
quadrupole has become the most widely used in water and
wastewater analysis.
The mass spectrometer detects compounds by ionizing mole-
cules into charged species with a 70-eV beam. The ions are
accelerated toward the quadrupole mass filter through a series of
lenses held at 0 to 200 V. The differently sized, charged frag-
ments are separated according to mass-to-charge ratio (related to
molecular weight) by means of the quadrupole, which uses
varying electric and radiofrequency (rf) fields. The quadrupole is
connected to a computer, which varies these fields so that only
fragments of one particular mass-to-charge ratio (⫾0.5) can
traverse the quadrupole at any one time. As the ions leave the
quadrupole they are attracted to the electron multiplier through
an electrical potential of several thousand volts. The charge
fragments, in turn, are detected by the electron multiplier. Be-
cause the electric and the rf fields are cycled every few seconds,
a fragmentation pattern is obtained. Each cycle is called a mass
scan. Most chemicals have unique fragmentation patterns, called
mass spectra. The computer contains, and can search, a library of
known mass spectra to identify tentatively an unknown com-
pound exhibiting a particular spectrum. Use authentic com-
pounds for confirmation after tentative identifications are made.
Background mass interference can result from the ability of the
mass spectrometer to detect any ions created in its ion volume (up
to a specified mass). Any compounds continuously present in the
source will be detected. Some mass ions always present are due to
air components that leak into the system, such as oxygen (masses 16
and 32), nitrogen (masses 14 and 28), carbon dioxide (mass 44),
argon (mass 40), and water (mass 18), or to helium carrier gas
(masses 4 and 8), or to diffusion pump oil vapors.
3.
High-Performance Liquid Chromatographic (HPLC)
Methods
a. Principle: HPLC is an analytical technique in which a liquid
mobile phase transports a sample through a column containing a
liquid stationary phase. The interaction of the sample with the
stationary phase selectively retains individual compounds and
permits separation of sample components. Detection of the sep-
arated sample compounds is achieved mainly through the use of
absorbance detectors for organic compounds and through con-
ductivity and electrochemical detectors for metal and inorganic
components.
INTRODUCTION (6010)/Analytical Methods
5
INTRODUCTION (6010)/Analytical Methods