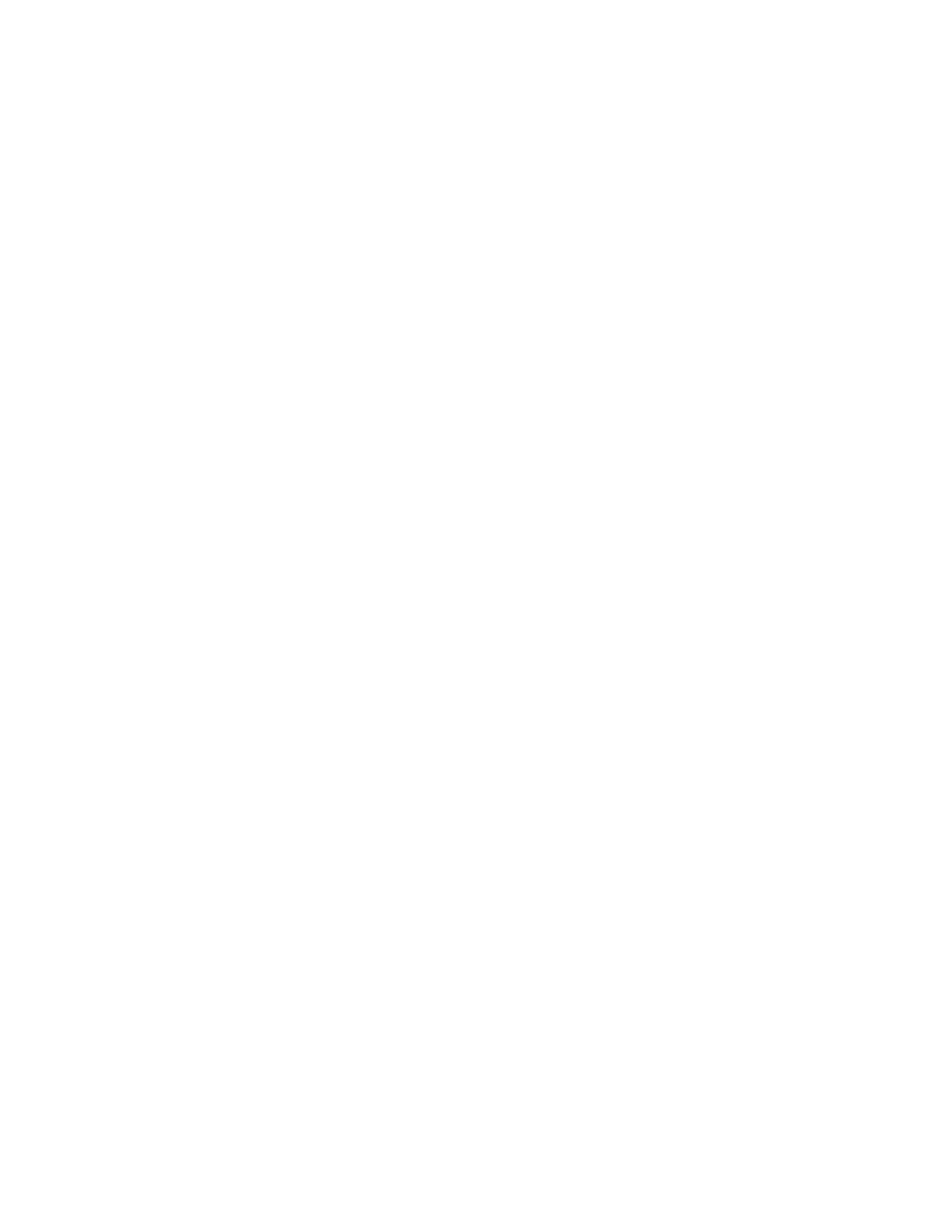
3. MCLAREN, J.W., A.P. MYKYTIUK, S.N. WILLIE & S.S. BERMAN. 1985.
Determination of trace metals in seawater by inductively coupled
plasma mass spectrometry with preconcentration on silica-immobil-
ized 8-hydroxyquinoline. Anal. Chem. 57:2907.
4. BURBA, P. & P.G. WILLMER. 1987. Multielement preconcentration
for atomic spectroscopy by sorption of dithiocarbamate metal com-
plexes (e.g., HMDC) on cellulose collectors. Fresenius Z. Anal.
Chem. 329:539.
5. WANG, X. & R.M. BARNES. 1989. Chelating resins for on-line flow
injection preconcentration with inductively coupled plasma atomic
emission spectroscopy. J. Anal. Atom. Spectrom. 4:509.
6. SIRIRAKS, A., H.M. KINGSTON & J.M. RIVIELLO. 1990. Chelation ion
chromatography as a method for trace elemental analysis in com-
plex environmental and biological samples. Anal. Chem. 62:1185.
7. PUGET SOUND WATER QUALITY AUTHORITY. 1996. Recommended
Guidelines for Measuring Metals in Puget Sound Marine Water,
Sediment and Tissue Samples. Appendix D: Alternate Methods for
the Analysis of Marine Water Samples. Puget Sound Water Quality
Authority, Olympia, Wash.
8. NELMS, S., ED. 2005. Inductively Coupled Plasma Mass Spectrom-
etry Handbook. Blackwell Science, Cambridge, Mass.
9. BECKER, J.S. 2008. Inorganic Mass Spectrometry: Principles and
Applications. Wiley Interscience, Malden, Mass.
10. U.S. ENVIRONMENTAL PROTECTION AGENCY. 1994. Determination of
trace elements in waters and wastes by inductively coupled plasma–
mass spectrometry, Method 200.8. U.S. Environmental Protection
Agency, Environmental Monitoring Systems Laboratory, Cincin-
nati, Ohio.
11. LONGBOTTOM, J.E., T.D. MARTIN, K.W. EDGELL, S.E. LONG, M.R.
PLANTZ & B.E. WARDEN. 1994. Determination of trace elements in
water by inductively coupled plasma–mass spectrometry: collabor-
ative study. J. AOAC Internat. 77:1004.
12. U.S. ENVIRONMENTAL PROTECTION AGENCY. 1995. Method 1638: De-
termination of trace elements in ambient waters by inductively
coupled plasma–mass spectrometry. U.S. Environmental Protection
Agency, Off. Water, Washington, D.C.
3125 B. Inductively Coupled Plasma–Mass Spectrometry (ICP–MS) Method
1.
General Discussion
a. Principle: In this method, analysts introduce sample ma-
terial to an argon-based, high-temperature radio-frequency
plasma, usually via pneumatic nebulization. As energy transfers
from the plasma to the sample stream, the target element des-
olves, atomizes, and ionizes. The resulting ions are extracted
from the plasma through a differential vacuum interface and
separated based on their mass-to-charge (m/z) ratio by a mass
spectrometer. Typically, either a quadrupole (with or without
CCT or DRC) or magnetic sector (high-resolution) mass spec-
trometer is used. An electron multiplier detector counts the
separated ions, and a computer-based data-management system
processes the resulting information.
b. Applicable elements and analytical limits: This method has
been demonstrated to be suitable for aluminum, antimony, arse-
nic, barium, beryllium, cadmium, chromium, cobalt, copper,
lead, manganese, molybdenum, nickel, selenium, silver, stron-
tium, thallium, uranium, vanadium, and zinc (see Table 3125:
III). It is also acceptable for other elemental analytes as long as
the same quality assurance practices are followed, with docu-
mented acceptance limits.
Before implementing the method, determine the instrument
detection limit (IDL) and method detection limit (MDL) for all
analytes (see Section 1030C). Typical IDLs for method analytes
are presented in Table 3125:III.
1,2
Determining the MDL for each element is critical when ana-
lyzing complex matrices, such as seawater, brines, and industrial
effluents. In these cases, the MDL typically will be higher than
the IDL because of background levels of analytes introduced
during sample preparation, as well as laboratory-derived con-
tamination and matrix-based interferences. Determine both IDL
and MDL when first implementing this method, and then repeat
yearly or whenever the instrument configuration changes or
major maintenance occurs, whichever happens first.
Also, determine the linear dynamic range (LDR) for all
method analytes, including multi-element mixtures (to account
for possible inter-element effects). LDR is the maximum analyte
concentration above the highest calibration point at which the
analyte response is within ⫾10% of its theoretical response.
When determining LDRs, avoid using unnecessarily high analyte
concentrations because they might damage the detector. Deter-
mine LDR when first implementing this method, and then repeat
yearly.
c. Interferences: ICP–MS is subject to several types of inter-
ferences, including the following:
1) Isobars—Isobars are isotopes of different elements that
form ions with the same nominal atomic mass units/charge
number (m/z) ratio that cannot be resolved by a quadrupole or
high-resolution mass spectrometer. Typically, ICP–MS operat-
ing software includes all known isobaric interferences and will
perform the necessary calculations automatically (see Table
3125:IV). Monitor
83
Kr,
99
Ru,
118
Sn, and
125
Te to correct for
isobaric interference caused by
82
Kr on
82
Se, by
98
Ru on
98
Mo,
by
114
Sn on
114
Cd, by
115
Sn on
115
In, and by
123
Te on
123
Sb.
Monitor ArCl at mass 77 to estimate chloride interferences.
Verify that all elemental and molecular correction equations
used in this method are correct and appropriate for the mass
spectrometer used and the sample matrix.
2) Abundance sensitivity—Abundance sensitivity is the possi-
bility that the low and high “wings” of any abundant mass peak
will contribute to or obscure adjacent masses. Adjust the mass
spectrometer resolution and quadrupole pole bias to minimize
these interferences.
3) Polyatomics—Polyatomics are (molecular) ion interfer-
ences caused by ions with more than one atom that have the
same nominal m/z ratio as the isotope of interest. Most of the
common molecular ion interferences have been identified (see
Table 3125:V). Because of the severity of chloride ion interfer-
ence on important analytes, particularly vanadium and arsenic,
hydrochloric acid is not recommended for use in ICP–MS sam-
INDUCTIVELY COUPLED PLASMA–MASS SPECTROMETRY (3125)/ICP–MS Method
2
INDUCTIVELY COUPLED PLASMA–MASS SPECTROMETRY (3125)/ICP–MS Method