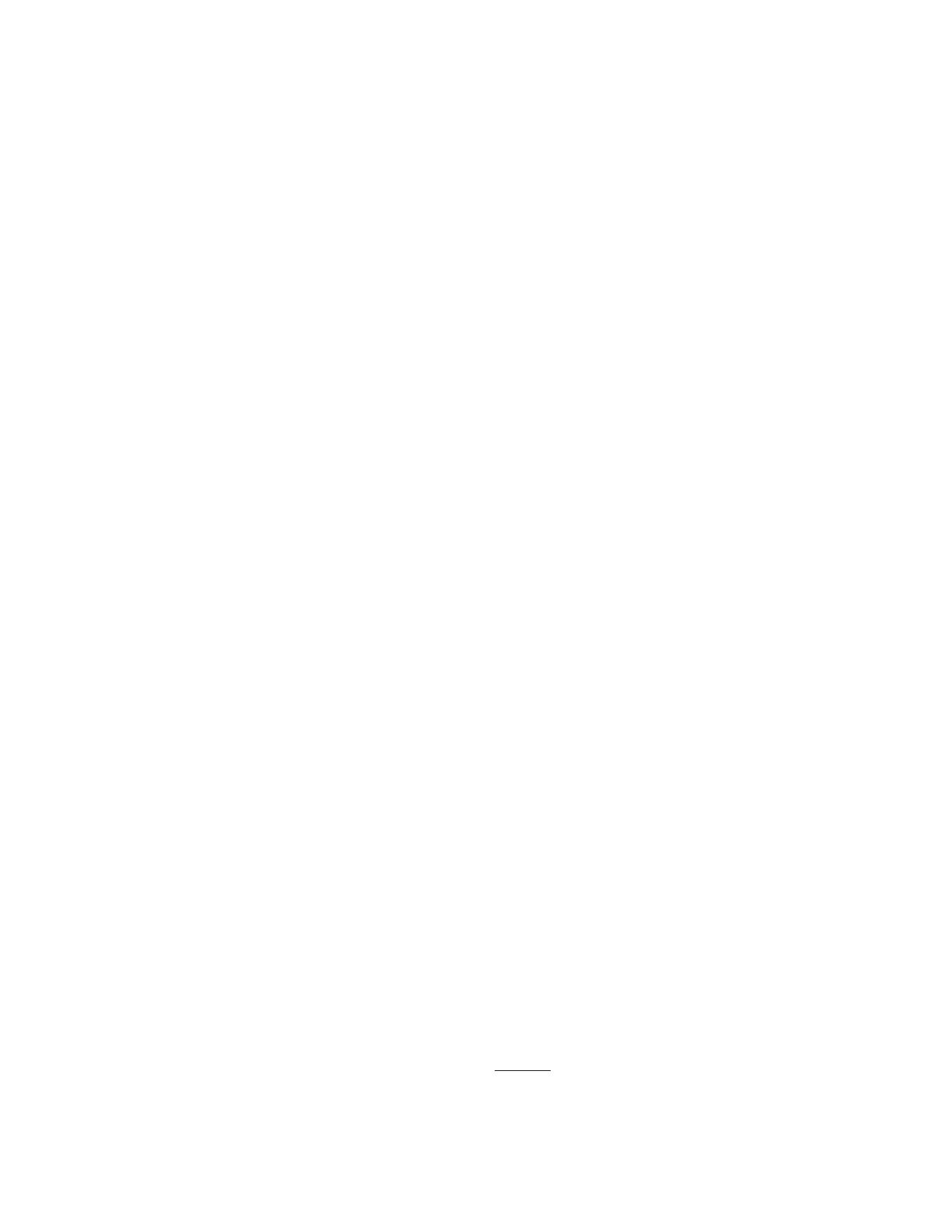
7010 INTRODUCTION*
7010 A. General Discussion
1.
Occurrence and Monitoring
Radioactivity in water and wastewater originates from both
natural sources and human activities (e.g., nuclear fuel-related
operations, from mining to reprocessing; medical uses of radio-
isotopes; industrial uses of radioisotopes; worldwide fallout from
atmospheric testing of nuclear devices; and enhanced concentra-
tion of naturally occurring radionuclides). Water and wastewater
monitoring programs should be designed to realistically assess
the degree of radioactive contamination. In some cases (e.g.,
compliance monitoring for drinking water), the conditions are
clearly defined.
1
In others, it may be necessary to examine the
individual situation
2
to determine the critical radionuclide(s), the
critical pathway by which such radionuclide(s) move(s) through
the environment, and a critical population group that is exposed
as a result. This approach will help narrow the list of possible
radionuclides to monitor.
A relevant list of the most hazardous radionuclides can be
created by examining the radioactivity concentration standards
issued by the International Committee on Radiation Protection
(ICRP),
3
the Federal Radiation Council (FRC),
4
the National
Committee on Radiation Protection and Measurement (NCRP),
2
the U.S. Environmental Protection Agency,
1
and agencies in
other countries. Applicable regulatory standards and action lev-
els may vary from state to state in the USA, as well as from
country to country.
Monitoring programs should provide both assurances when
environmental conditions are safe and adequate warning when
they are not so proper precautions can be taken. Therefore, it is
necessary to establish baselines for naturally occurring radionu-
clides (kinds and quantities) so changes can be measured. This
will help decision-makers make sound judgments on the hazard-
ous or nonhazardous nature of increased concentrations.
2.
Types of Measurement
Meaningful measurements require careful application of good
scientific techniques. The types of measurements to be made
depend on the testing objectives. Gross alpha and gross beta
screening measurements are relatively inexpensive, can be com-
pleted quickly, and are useful for determining whether further
analysis for specific radionuclides may be merited. However,
they do not provide information about the sample’s isotopic
composition, cannot be used to estimate radiation dose, and are
subject to significant bias if the sample contains other radionu-
clides or has a high concentration of dissolved solids. While
calibration standards that closely match the samples’ character-
istics and carefully applied self-absorption and cross-talk correc-
tions may help minimize bias and optimize precision, gross
activity measurements are generally not capable of providing
reliable, unbiased results of known precision that reflect the
samples’ isotopic makeup.
Specific radionuclide measurements must be made if dose
estimates are needed [e.g., when gross analyses
1
results exceed
established action limits (regulatory or other) or long-term trends
are being monitored]. They usually are more expensive and
time-consuming than a gross analysis. Specific measurements
identify radionuclides by the type and energy of emitted radia-
tion, chemical techniques, half-life, or some combination of
these. Gamma-emitting radionuclides can be measured rapidly
and with minimal sample preparation via gamma spectrometry.
Chemical separations make it possible to improve measurement
results by increasing the quantity of sample that can be measured
and decreasing interference from non-target radionuclides in
samples.
Knowing the chemical and radiochemical characteristics of
the measured radionuclide is critical for satisfactory results.
Gross alpha and gross beta results cannot provide accurate
information about radionuclides whose energies are significantly
different from those of the calibration standard. When concen-
trating water samples via evaporation, certain radionuclides
(e.g., radioiodine, polonium, tritium, and carbon-14) may be lost
to volatilization. If the sample is ignited, the chance of volatil-
ization loss is even greater. When analyzing samples containing
uranium and thorium, keep in mind that members of these series
are rarely present in secular equilibrium. Identifying short-lived
radionuclides, which may not be in secular equilibrium during
sampling, may require that samples be analyzed shortly after
collection and that analysts consider the dynamic nature of
radionuclide concentrations as a result of decay and ingrowth
phenomena.
3. References
1. U.S. ENVIRONMENTAL PROTECTION AGENCY. 2000. National primary
drinking water regulations; radionuclides; final rule. 40 CFR Parts 9,
141 & 142. Fed. Reg. 65(236):76708.
2. U.S. ENVIRONMENTAL PROTECTION AGENCY. 2004. National primary
drinking water regulations: analytical method for uranium. Fed. Reg.
69(164):52176.
3. U.S. ENVIRONMENTAL PROTECTION AGENCY. 2002. Implementation
Guidance for Radionuclides, EPA 816-F-00-002. U.S. Environmen-
tal Protection Agency, Off. Ground Water and Drinking Water,
Washington, D.C. Available at http://water.epa.gov/lawsregs/rules-
regs/sdwa/radionuclides/compliancehelp.cfm. Accessed August
2011.
4. NATIONAL COMMITTEE on RADIATION PROTECTION and MEASUREMENTS.
1959. Maximum Permissible Body Burdens and Maximum Permis-
sible Concentrations of Radionuclides in Air and Water for Occupa-
tional Exposure, NBS Handbook No. 69, pp. 1, 17, 37, 38 & 93.
National Committee on Radiation Protection and Measurements,
Bethesda, Md.
* Approved by Standard Methods Committee, 2011.
Joint Task Group: Robert T. Shannon (chair), Shiyamalie R. Ruberu, Bahman
Parsa.
10-1