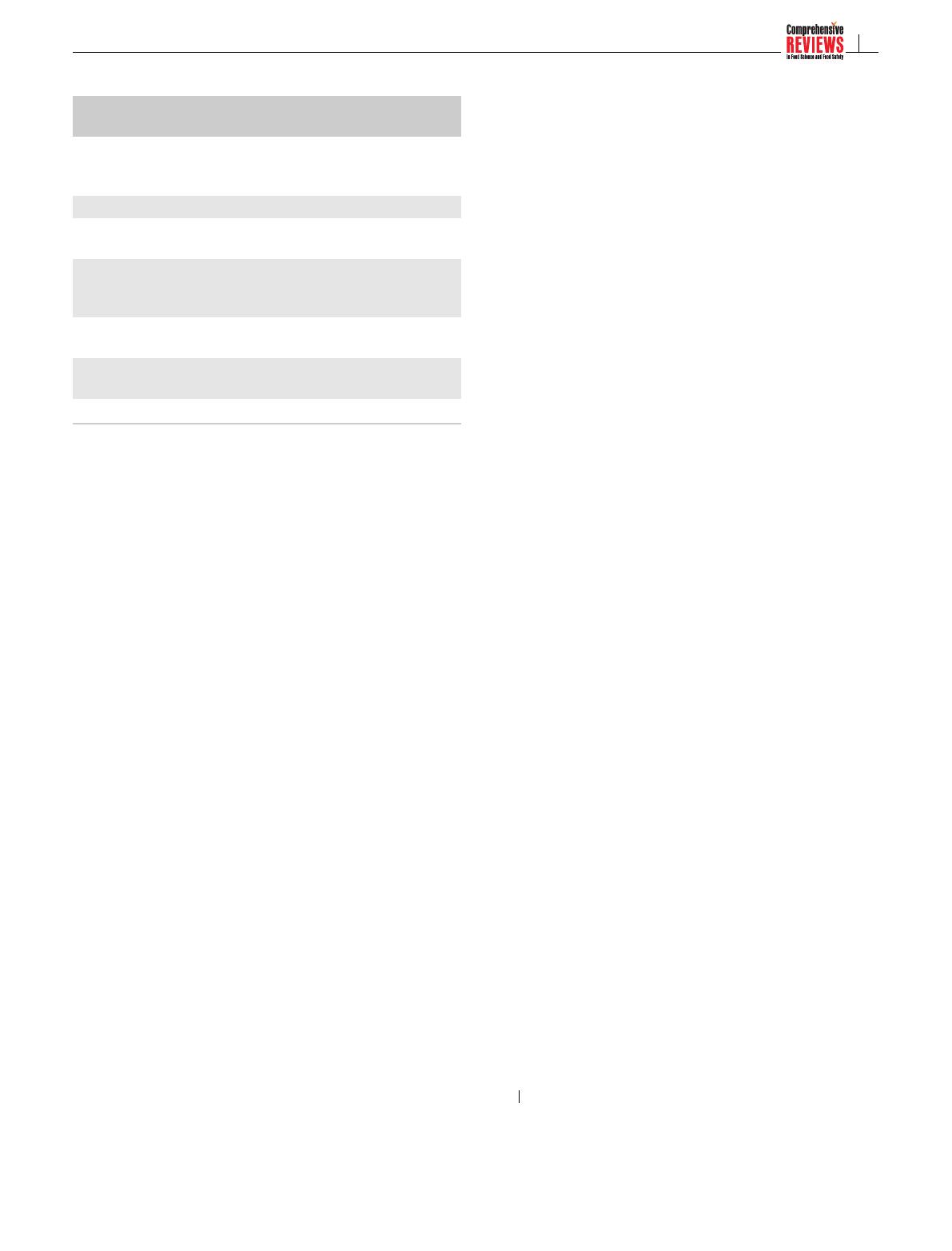
PROGRESS IN MICELLAR CASEIN CONCENTRATE: PRODUCTION AND APPLICATIONS 3
TABLE 1 The functional properties of milk casein (CN)
Functional
properties Casein (CN)
Hydration High water binding capacity
at higher concentration gels
Minimum at the isoelectric point (pH =4.6)
Solubility Insoluble at the isoelectric point (pH =4.6)
Viscosity Low viscosity at the isoelectric point (pH =4.6)
A viscous solution at neutral and alkaline pH
Gelation Micelle gelation by rennet enzyme
No thermal gelation except in the presence of
calcium
Emulsifying
properties
Excellent emulsifying properties at neutral and
alkaline pH
Foaming
properties
Good foaming properties and overrun but low
foam stability
Flavor binding Good flavor binding
Adapted from Lorient et al. (1991).
CN from the serum with Pasteur-Chamberland porcelain
filters. Subsequently, the colloidal particles were found
to contain calcium phosphate. In 1910, it was hypothe-
sized that CN exists in a protective colloidal model, where
the CN (irreversible unstable colloid) was covered by lac-
talbumin (reversible stable colloid). This hypothesis was
then examined by coagulating bovine, human, and don-
key’s milk with rennet (Alexander, 1910; Alexander &
Bullowa, 1910). The produced gel from human and don-
key’s milk was very weak as compared to bovine milk
because of the lower CN and higher lactalbumin. Wieg-
ner (1914) described the physical state of CN as large par-
ticles associated together and disassembled them by dis-
placing the calcium with sodium. Subsequently, Duclaux
(1920) described macroclusters of CN as micelle.
Palmer and Richardson (1925) rejected the protective
colloidal model, and postulated the precipitation of CN due
to the cations. Palmer and Richardson coined a new termi-
nology for the casein micelle, including colloidal calcium
caseinate, calcium caseinate, or calcium caseinate-calcium
phosphate. Concomitantly, Sorensen (1930)employedthe
term “component system” to describe the CN macroclus-
ters instead of the “micelle” term.
During that period, the isolation of the CN structure was
a significant challenge. Pedersen (1936) ultracentrifuged
milk to obtain CN and studied its polydispersity. Peder-
son’s development represented a significant milestone to
make it possible to study different characteristics of CN
in milk. Years later, Langmuir and Waugh (1940) reported
the hydrophilic and hydrophobic properties of CN. Dur-
ing the 1950s, the term “casein micelle” was widely used to
describe the state of milk CN (Pyne, 1953), where noncova-
lent forces maintained the protein association and aggre-
gation (Waugh, 1954).
The aggregation of the CN network was an active area
of research throughout 1960s. The roles of pH, tempera-
ture, and protein content were extensively evaluated (von
Hippel & Waugh, 1955). Waugh and Von Hippel (1956)
invented a methodology to isolate the κ-CN that allowed
an in-depth study of the CN structure. In 1957, the κ-CN
was found to be about 12-15% of the CN content when the
calcium was soluble, and 10 times more when the micelle
was aggregated, reaching a size up to 2000 Å with an aver-
age of 1000 Å (Fox & Foster, 1957). After that, many stud-
ies were performed on the structure of the CN micelle. In
1958, the first microscopy study of the CN was reported
(Barbaro & Calapuj, 1958),whichmakeitpossibletopro-
pose the first CN micelle model (Waugh, 1958). Before the
1960s, CN was manufactured for industrial applications
(e.g., plastic, paints, and glues). After the 1960s, Australia
and New Zealand initiated to utilize CN as a food ingre-
dient. In 1972, researchers studied some functional char-
acteristics of CN such as viscosity and surface tension in
CN solutions of buffalo and cow milk (Puri et al., 1972).
Badertscher and Chaveron (1978) have invented a process
to produce CN and caseinates in 1978. Ten years later, the
emulsifying characteristics of CN micelle were studied in
bovine milk (Haque et al., 1988). Years later, in 1992, alter-
native models to submicelles associated together by cal-
cium phosphate complexes (CCP) were proposed (Visser,
1992). At the beginning of 1990, MF has been used in the
dairy industry to reduce the bacterial count, as well as, frac-
tionation of CN and SP without heat treatment or chemi-
cals, which keep the CN in its native status (Haeusl et al.,
1990; Olesen et al., 1990). In 1994, MF has applied to exam-
ine the phage distribution in permeate and retentate pro-
duced from skim milk (Gautier et al., 1994). The protein
composition of MCC produced from MF was studied in
1999 (Jost et al., 1999). Since then, MF has been applied
to milk to produce many dairy products, especially cheese
(Garem et al., 2000; Lidberg & Bredahl, 1990). CN has dif-
ferent characteristics and a wide range of applications; so
many researchers are still studying and will continue to
examine these properties. The CN is widely used nowa-
days as a functional food ingredient (Huppertz et al., 2004).
As a result, Guinee et al. (2009)havepatentedMCCpow-
ders with different ratios of calcium to CN for making
cheese. Between 2000 and 2008, the number of new prod-
ucts containing CN grew by 22% in the United State annu-
ally (Affertsholt, 2009) and expected to have a continual
increase in the future.
3 STRUCTURE OF CASEIN MICELLE
The CN structure in bovine milk is composed of several
protein fractions (αS1,αS2,β,andκ-CN which presents