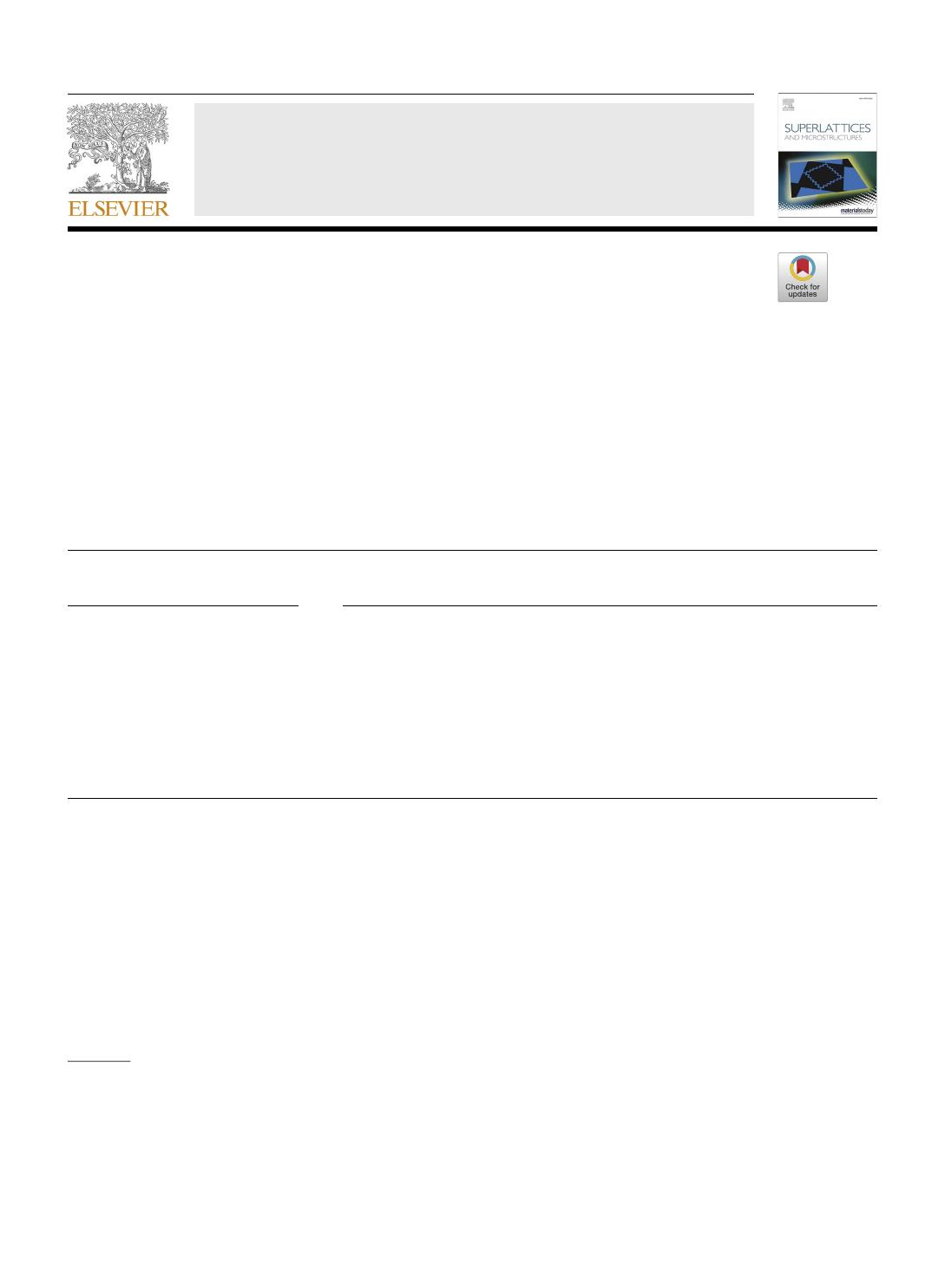
Superlattices and Microstructures 142 (2020) 106473
Available online 28 February 2020
0749-6036/© 2020 Elsevier Ltd. All rights reserved.
Impact of substrate nature and lm thickness on physical
properties of antimony trisulphide (Sb
2
S
3
) thin lms for
multifunctional device applications
S. Ben Ameur
a
,
b
,
*
, B. Duponchel
c
, G. Leroy
b
, H. Maghraoui-Meherzi
d
, M. Amlouk
e
,
H. Guermazi
a
, S. Guermazi
a
a
Research Unit: Physics of Insulators and Semi Insulator Materials, Faculty of Science of Sfax, Road of Soukra Km 3.5, B.P: 1171 3000, Sfax,
University of Sfax, Tunisia
b
Unit�
e de Dynamique et Structure des Mat�
eriaux Mol�
eculaires (UDSMM) EA 4476, Universit�
e du Littoral C^
ote d’Opale, 62228, Calais, France
c
Unit�
e de Dynamique et Structure des Mat�
eriaux Mol�
eculaires (UDSMM) EA 4476, Universit�
e du Littoral C^
ote d’Opale, 59140, Dunkerque, France
d
Laboratoire de Chimie Analytique et Electrochimie, Faculty of Science of Tunis, Tunis El Manar University, Tunisia
e
Research Unit: Physics of Semi-conductor devices, Faculty of Science of Tunis, Tunis El Manar University, 2092, Tunis, Tunisia
ARTICLE INFO
Keywords:
Antimony trisulphide (Sb
2
S
3
)
Film thickness
Flexible polymer substrate
Hydrophobic surfaces
ABSTRACT
Sb
2
S
3
thin lms were deposited with various thickness on glass and exible polymer Poly-
etherimide (PEI) substrates by simple chemical bath deposition. X-ray diffraction patterns reveal
the formation of polycrystalline lms with orthorhombic structure. The Sb
2
S
3
thin lms grown on
the PEI substrate are rougher. The enhancement of roughness for Sb
2
S
3
/PEI thin lms leads to
improve their hydrophobic character. Furthermore, they show high values of contact angle (CA)
around 162. The absorption coefcient of thin lms is determined to be higher than 10
4
cm
1
.
The band-gap energy has been found to be between 1.6 and 2.1 eV as a function of thickness
which related to the variation of structural defects. Thus, the growth of these lms signicantly
depends on the nature of the substrate and its thickness. The obtained results show that all Sb
2
S
3
on glass and PEI thin lms have potential applications in the self-cleaning windows and solar
cells.
1. Introduction
The fabrication of thin lms for various devices like sensors, solar cells, light-emitting diodes has received immense interest of
scientic community since many years. In general, thin lms are deposited on amorphous substrate (i.e. glass). To overcome the
overweight and rigidity of conventional glass substrates, we have observed an increasing interest in the choice of a specic substrate
that depends on the temperature of deposition and post deposition processing. The use of polymer as a substrate can be introduced in
the preparation of exible displays and electronic devices [1–3]. Recently, the deposition of metal oxides, like ZnO [4,5] and SnO
2
[6],
on a exible substrate has been reported which has received remarkable industrial applications of such devices. Additionally, several
polymers such as polyethylene terephthalate (PET) [7] and polyethylene naphthalate (PEN) [4] are also used as exible polymer
substrates.
* Corresponding author. Faculty of Science of Sfax, B.P: 1171 3038, Sfax, University of Sfax, Tunisia.
Contents lists available at ScienceDirect
Superlattices and Microstructures
journal homepage: www.elsevier.com/locate/superlattices
https://doi.org/10.1016/j.spmi.2020.106473
Received 18 November 2019; Received in revised form 25 January 2020; Accepted 27 February 2020