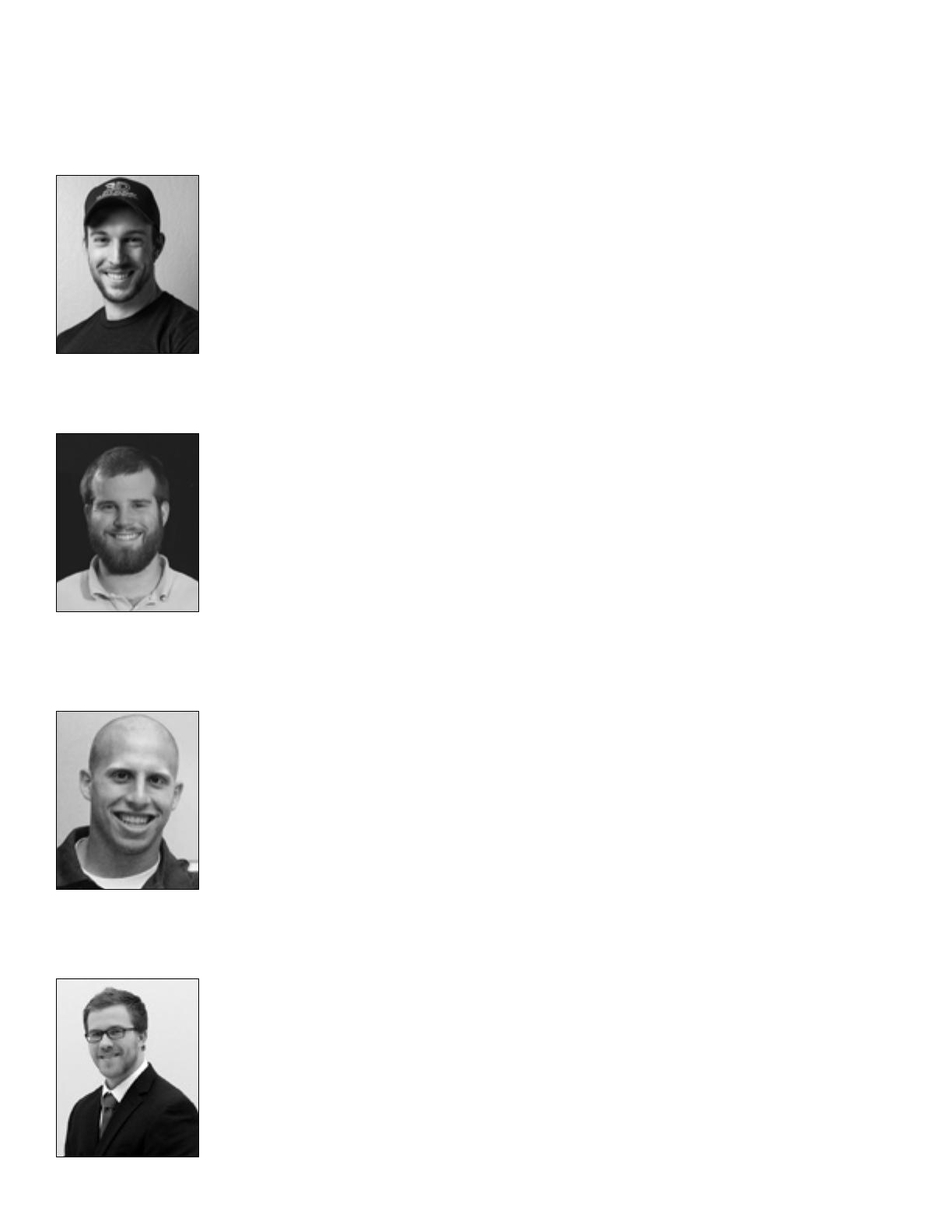
The Reviewers
Eric Helms
Eric Helms is a coach, athlete, author, and educator. He is a coach for drug-free strength and physique
competitors at all levels as a part of team 3D Muscle Journey where he is also the Chief Science
Ocer. Eric regularly publishes peer-reviewed articles in exercise science and nutrition journals on
physique and strength sport, in addition to contributing to the 3DMJ blog. He’s taught undergraduate-
and graduate-level nutrition and exercise science and speaks internationally at academic and
commercial conferences. He has a B.S. in tness and wellness, an M.S. in exercise science, a second
Master’s in sports nutrition, a Ph.D. in strength and conditioning, and is a research fellow for the Sports
Performance Research Institute New Zealand at Auckland University of Technology. Eric earned pro
status as a natural bodybuilder with the PNBA in 2011 and competes in numerous strength sports.
Greg Nuckols
Greg Nuckols has over a decade of experience under the bar and a B.S. in exercise and sports
science. Greg earned his M.A. in exercise and sport science from the University of North Carolina
at Chapel Hill. He’s held three all-time world records in powerlifting in the 220lb and 242lb classes.
He’s trained hundreds of athletes and regular folks, both online and in-person. He’s written for many
of the major magazines and websites in the tness industry, including Men’s Health, Men’s Fitness,
Muscle & Fitness, Bodybuilding.com, T-Nation, and Schwarzenegger.com. Furthermore, he’s had the
opportunity to work with and learn from numerous record holders, champion athletes, and collegiate
and professional strength and conditioning coaches through his previous job as Chief Content
Director for Juggernaut Training Systems and current full-time work on StrongerByScience.com.
Michael C. Zourdos
Michael (Mike) C. Zourdos, Ph.D., CSCS, has specializations in strength and conditioning and skeletal
muscle physiology. He earned his Ph.D. in exercise physiology from The Florida State University (FSU)
in 2012 under the guidance of Dr. Jeong-Su Kim. Prior to attending FSU, Mike received his B.S. in
exercise science from Marietta College and M.S. in applied health physiology from Salisbury University.
Mike served as the head powerlifting coach of FSU’s 2011 and 2012 state championship teams. He
also competes as a powerlifter in the USAPL, and among his best competition lifts is a 230kg (507lbs)
raw squat at a body weight of 76kg. Mike owns the company Training Revolution, LLC., where he has
coached more than 100 lifters, including a USAPL open division national champion.
Eric Trexler
Eric Trexler is a pro natural bodybuilder and a sports nutrition researcher. Eric has a PhD in Human
Movement Science from UNC Chapel Hill, and has published dozens of peer-reviewed research
papers on various exercise and nutrition strategies for getting bigger, stronger, and leaner. In
addition, Eric has several years of University-level teaching experience, and has been involved in
coaching since 2009. Eric is the Director of Education at Stronger By Science.