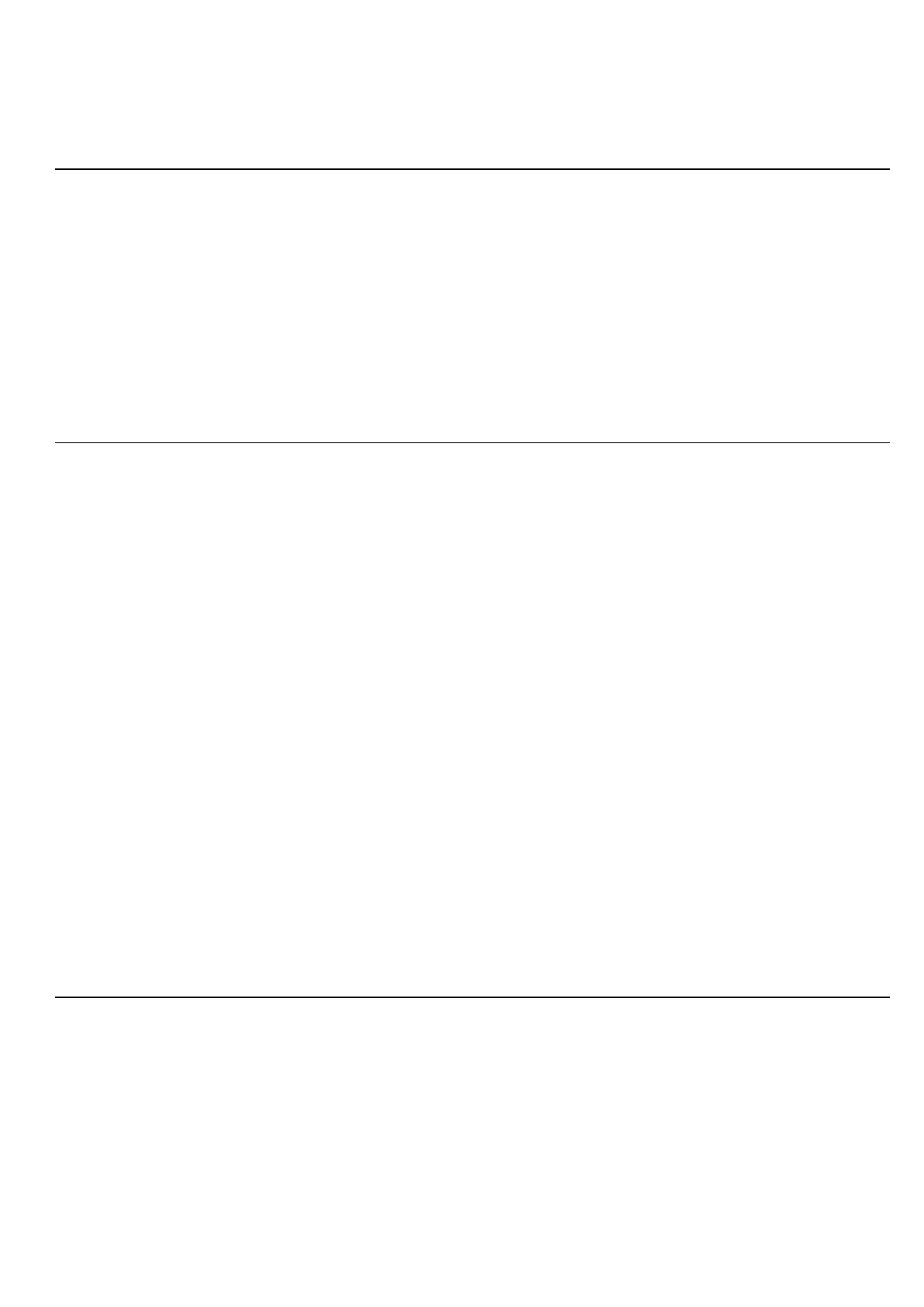
Sommaire
Introduction --------------------------------------------------------------------------------------------------------------------------------------------- 1
La maitrise de la qualité, un enjeu pour la commercialisation de la mangue. ----------------------------------------------------------------------------------- 2 I -
La qualité de la mangue : de nombreux attributs qui varient au cours de la croissance et de la maturation du fruit. ------------------------------- 4 II -
Les facteurs influençant la qualité et le mûrissement de la mangue : synthèse bibliographique. -------------------------------------------------------- 8 III -
A - Le bilan énergétique du fruit varie en fonction de son micro environnement. ------------------------------------------------------------------------------- 8
B - Le bilan hydrique du fruit est lié à son bilan carboné et énergétique. ------------------------------------------------------------------------------------------ 9
C - Le bilan carboné de la mangue varie en fonction de la charge en fruits -------------------------------------------------------------------------------------- 10
D - Conclusions de la synthèse bibliographique------------------------------------------------------------------------------------------------------------------------- 11
E - Plan de travail --------------------------------------------------------------------------------------------------------------------------------------------------------------- 13
Partie I : Hétérogénéité de la qualité et de la maturité au sein de la mangue ------------------------------------------------------ 14
Non-destructive prediction of color and pigment contents in mango peel ------------------------------------------------------------------------------------- 16 I -
A - Abstract----------------------------------------------------------------------------------------------------------------------------------------------------------------------- 17
B - Introduction ----------------------------------------------------------------------------------------------------------------------------------------------------------------- 18
C - Materials and methods --------------------------------------------------------------------------------------------------------------------------------------------------- 19
D - Results and discussion ---------------------------------------------------------------------------------------------------------------------------------------------------- 23
Model-assisted analysis of spatial and temporal variations in fruit temperature and transpiration highlighting the role of fruit II -
development ------------------------------------------------------------------------------------------------------------------------------------------------------------------------ 34
A - Abstract----------------------------------------------------------------------------------------------------------------------------------------------------------------------- 35
B - Introduction ----------------------------------------------------------------------------------------------------------------------------------------------------------------- 36
C - Materials and methods --------------------------------------------------------------------------------------------------------------------------------------------------- 38
D - Results ------------------------------------------------------------------------------------------------------------------------------------------------------------------------ 48
E - Discussion -------------------------------------------------------------------------------------------------------------------------------------------------------------------- 59
Spatial and temporal variations in mango colour, acidity, and sweetness in relation to temperature and ethylene gradients within the III -
fruit ------------------------------------------------------------------------------------------------------------------------------------------------------------------------------------ 62
A - Abstract----------------------------------------------------------------------------------------------------------------------------------------------------------------------- 63
B - Introduction ----------------------------------------------------------------------------------------------------------------------------------------------------------------- 64
C - Materials and methods --------------------------------------------------------------------------------------------------------------------------------------------------- 65
D - Results ------------------------------------------------------------------------------------------------------------------------------------------------------------------------ 71
E - Discussion -------------------------------------------------------------------------------------------------------------------------------------------------------------------- 81
Partie II: Hétérogénéité de la qualité et de la maturité entre les mangues. --------------------------------------------------------- 85
Factors affecting ethylene and carbon dioxide concentrations during ripening: incidence on final dry matter, total soluble solids content I -
and acidity of mango fruit. ------------------------------------------------------------------------------------------------------------------------------------------------------ 87
A - Introduction ----------------------------------------------------------------------------------------------------------------------------------------------------------------- 88
B - Material and methods ---------------------------------------------------------------------------------------------------------------------------------------------------- 90
C - Results ------------------------------------------------------------------------------------------------------------------------------------------------------------------------ 95
D - Discussion ------------------------------------------------------------------------------------------------------------------------------------------------------------------ 103
Growing conditions and fruit detachment impacts on ethylene production: a modeling approach. ------------------------------------------------- 106 II -
A - Introduction --------------------------------------------------------------------------------------------------------------------------------------------------------------- 107