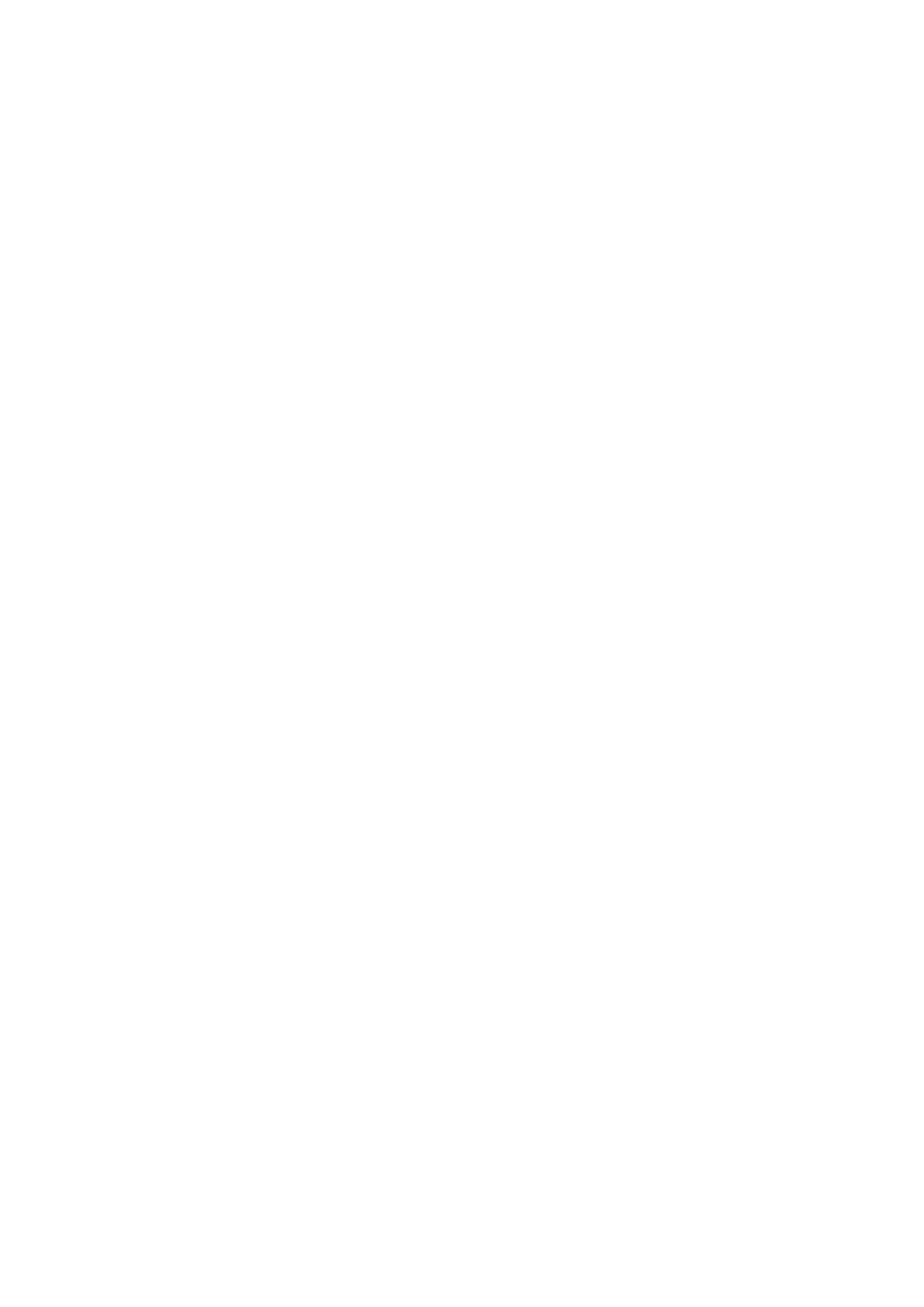
A Green Vitruvius – Principles and Practice of Sustainable Architectural Design
This edition was first published in 2011 by Earthscan
The first edition was published in 1999 by James & James (Science Publishers), and was prepared
within the THERMIE programme of the European Commission Energy Directorate, in a project
involving the UCD Energy Research Group, University College Dublin (Co-ordinators), the
Architects’ Council of Europe (ACE), Softech, Turin and the Finnish Association of Architects
(SAFA), Helsinki.
This second edition was fully revised and extended by Vivienne Brophy, University College Dublin
and J Owen Lewis (now with the Sustainable Energy Authority of Ireland), with assistance from
Ray McNally, Rory Walsh and Donal Finn. Graphic design by Sinead Kenny
Copyright © University College Dublin 2011
The material contained in this publication is presented in good faith, but its application must be
considered in the light of circumstances of individual projects. Neither University College Dublin,
the European Commission nor the contractors, agents or their subcontractors, or authors can be
held responsible for any effect, loss or expense resulting from the use of the material presented
in this publication.
The moral right of the authors has been asserted.
All rights reserved. No part of this publication may be reproduced, stored in a retrieval system, or
transmitted, in any form or by any means, electronic, mechanical, photocopying, recording or
otherwise, except as expressly permitted by law, without the prior, written permission of the
publisher.
Earthscan Ltd, Dunstan House, 14a St Cross Street, London EC1N 8XA, UK
Earthscan LLC, 1616 P Street, NW, Washington, DC 20036, USA
Earthscan publishes in association with the International Institute for Environment and
Development
For more information on Earthscan publications, see www.earthscan.co.uk or write to
earthinfo@earthscan.co.uk
ISBN: 978-1-84971-311-5 hardback
978-1-84971-191-3 paperback
Cover Image: GSW Headquarters, Berlin. Architect: Sauerbruch Hutton. Source: Christof Gessner.
A catalogue record for this book is available from the British Library
Library of Congress Cataloging-in-Publication Data
Brophy, Vivienne.
A green vitruvius : principles and practice of sustainable architectural design / Vivienne Brophy and
J. Owen Lewis.
p. cm.
Includes bibliographical references and index.
ISBN 978-1-84971-311-5 (hb) -- ISBN 978-1-84971-191-3 (pb) 1. Architectural design--
Technological innovations. 2. Architecture and society. 3. Buildings--Repair and reconstruction--
Standards. I. Lewis, J. Owen. II. Title.
NA2542.35.B78 2011
720'.47--dc22
2011003267
At Earthscan we strive to minimize our environmental impacts and carbon footprint through reducing
waste, recycling and offsetting our CO2emissions, including those created through publication of this
book. For more details of our environmental policy, see www.earthscan.co.uk.
Printed and bound in the UK
by Ashford Colour Press Ltd.
The paper used is FSC certified.
acknowledgements_03_acknowledgements.qxd 30/03/2011 20:47 Page ii