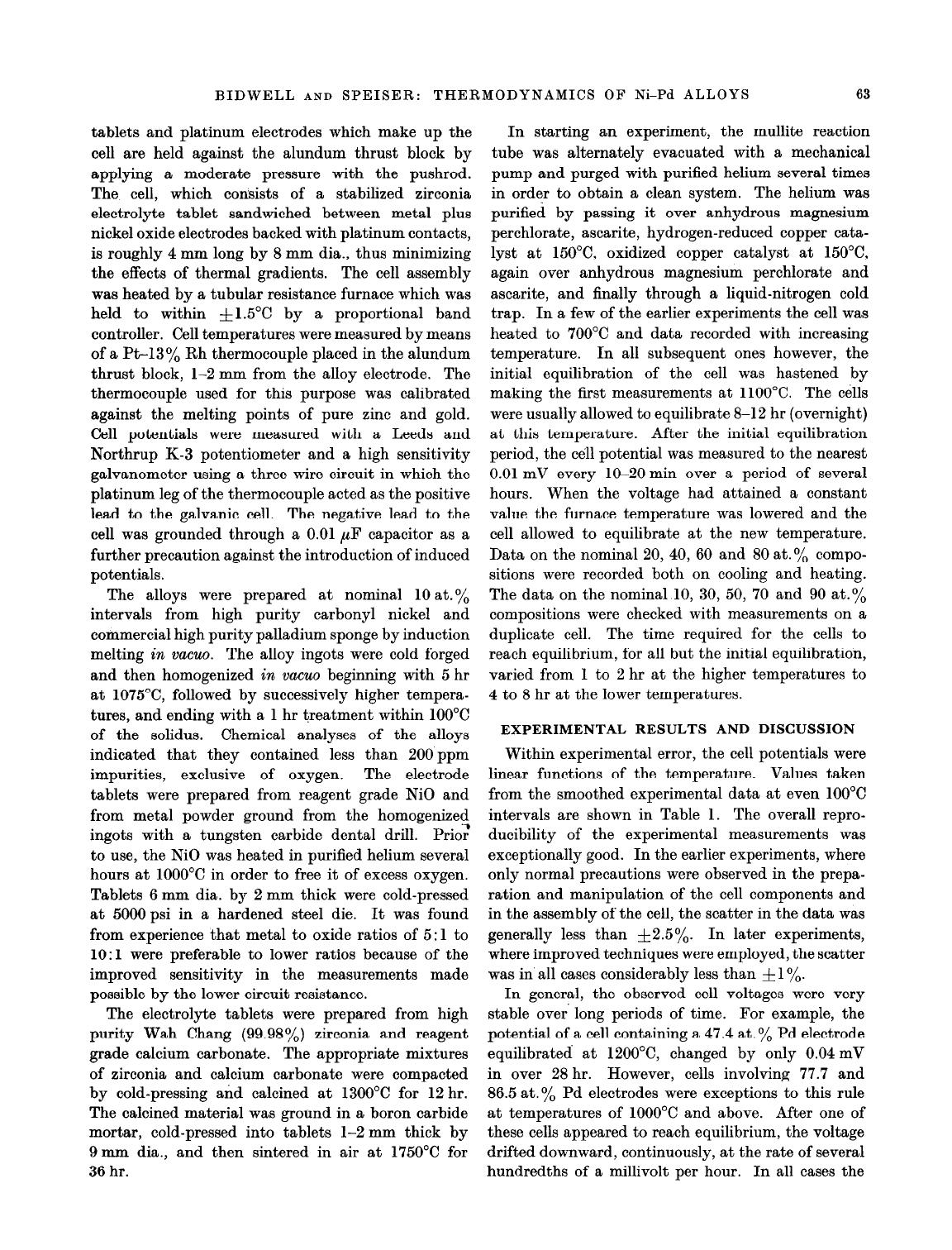
BIDWELL AND SPEISER: THERMODYNAMICS OF Ni-Pd ALLOYS 63
tablets and platinum electrodes which make up the
cell are held against the alundum thrust block by
applying a moderate pressure with the pushrod.
The cell, which consists of a stabilized zirconia
electrolyte tablet sandwiched between metal plus
nickel oxide electrodes backed with platinum contacts,
is roughly 4 mm long by 8 mm dia., thus minimizing
the effects of thermal gradients. The cell assembly
was heated by a tubular resistance furnace which was
held to within f 1.5”C by a proportional band
controller. Cell temperatures were measured by means
of a Pt-13% Rh thermocouple placed in the alunclum
thrust block, l-2 mm from the alloy electrode. The
thermocouple used for this purpose was calibrated
against the melting points of pure zinc and gold.
Cell potentials were measured with a Leeds and
Northrup K-3 potentiometer and a high sensitivity
galvanometer using a three wire circuit in which the
platinum leg of the thermocouple acted as the positive
lead to the galvanic cell. The negative lead to the
cell was grounded through a 0.01 ,uF capacitor as a
further precaution against the introduction of induced
potentials.
The alloys were prepared at nominal 10 at.%
intervals from high purity carbonyl nickel and
commercial high purity palladium sponge by induction
melting in vacua. The alloy ingots were cold forged
and then homogenized in vacua beginning with 5 hr
at 1075”C, followed by successively higher tempera-
tures, and ending with a 1 hr treatment within 100°C
of the solidus. Chemical analyses of the alloys
indicated that they contained less than 200 ppm
impurities, exclusive of oxygen. The electrode
tablets were prepared from reagent grade NiO and
from metal powder ground from the homogenized
ingots with a tungsten carbide dental drill. Prio;
to use, the NiO was heated in purified helium several
hours at 1000°C in order to free it of excess oxygen.
Tablets 6 mm dia. by 2 mm thick were cold-pressed
at 5000 psi in a hardened steel die. It was f0und
from experience that metal to oxide ratios of 5 : 1 to
10: 1 were preferable to lower ratios because of the
improved sensitivity in the measurements made
possible by the lower circuit resistance.
The electrolyte tablets were prepared from high
purity Wah Chang (99.98%) zirconia and reagent
grade calcium carbonate. The appropriate mixtures
of zirconia and calcium carbonate were compacted
by cola-pressing ad calcinecl at 1300°C for 12 hr.
The calcined material was ground in a boron carbide
mortar, cold-pressed into tablets 1-2 mm thick by
9 mm dia., and then sinterecl in air at 1750°C for
36 hr.
In starting an experiment, the mull&e reaction
tube was alternately evacuated with a mechanical
pump and purged with purifiecl helium several times
in order to obtain a clean system. The helium was
purified by passing it over anhydrous magnesium
perchlorate, ascarite, hydrogen-reduced copper cata-
lyst at 15O”C, oxidized copper catalyst at 15O”C,
again over anhydrous magnesium perchlorate and
ascarite, and finally through a liquid-nitrogen cold
trap. In a few of the earlier experiments the cell was
heated to 700°C and data recorded with increasing
temperature. In all subsequent ones however, the
initial equilibration of the cell was hastened by
making the first measurements at 1100°C. The cells
were usually allowed to equilibrate 8-12 hr (overnight)
at this temperature. After the initial equilibration
period, the cdl potential was measured to the nearest
0.01 mV every 10-20 min over a period of several
hours. When the voltage had attained a constant
value the furnace temperature was lowered and the
cell allowed to equilibrate at the new temperature.
Data on the nominal 20, 40, 60 and 80 at.% compo-
sitions were recorded both on cooling and heating.
The data on the nominal 10, 30, 50, 70 and 90 at.%
compositions were checked with measurements on a
duplicate cell. The time required for the cells to
reach equilibrium, for all but the initial equilibration,
varied from 1 to 2 hr at the higher temperatures to
4 to 8 hr at the lower temperatures.
EXPERIMENTAL RESULTS AND DISCUSSION
Within experimental error, the cell potentials were
linear functions of the temperature. Values taken
from the smoothed experimental data at even 100°C
intervals are shown in Table 1. The overall repro-
ducibility of the experimental measurements was
exceptionally good. In the earlier experiment,s, where
only normal precautions were observed in the prepa-
ration and manipulation of the cell components and
in the assembly of the cell, the scatter in the data was
generally less than &2.5%. In later experiments,
where improved techniques were employed, the scatter
was in all cases considerably less than f 1 Oh.
In general, the observed cell voltages were very
stable over’ long periods of time. For example, the
pot.ential of a cell containing a 47.4 at. y. Pa electrode
equilibrated at 12OO”C, changed by only 0.04 mV
in over 28 hr. However, cells involving 77.7 and
86.5 at.% Pd electrodes were exceptions to this rule
at temperatures of 1000°C and above. After one of
these cells appeared to reach equilibrium, the voltage
drifted downward, continuously, at the rate of several
hundredths of a millivolt per hour. In all cases the