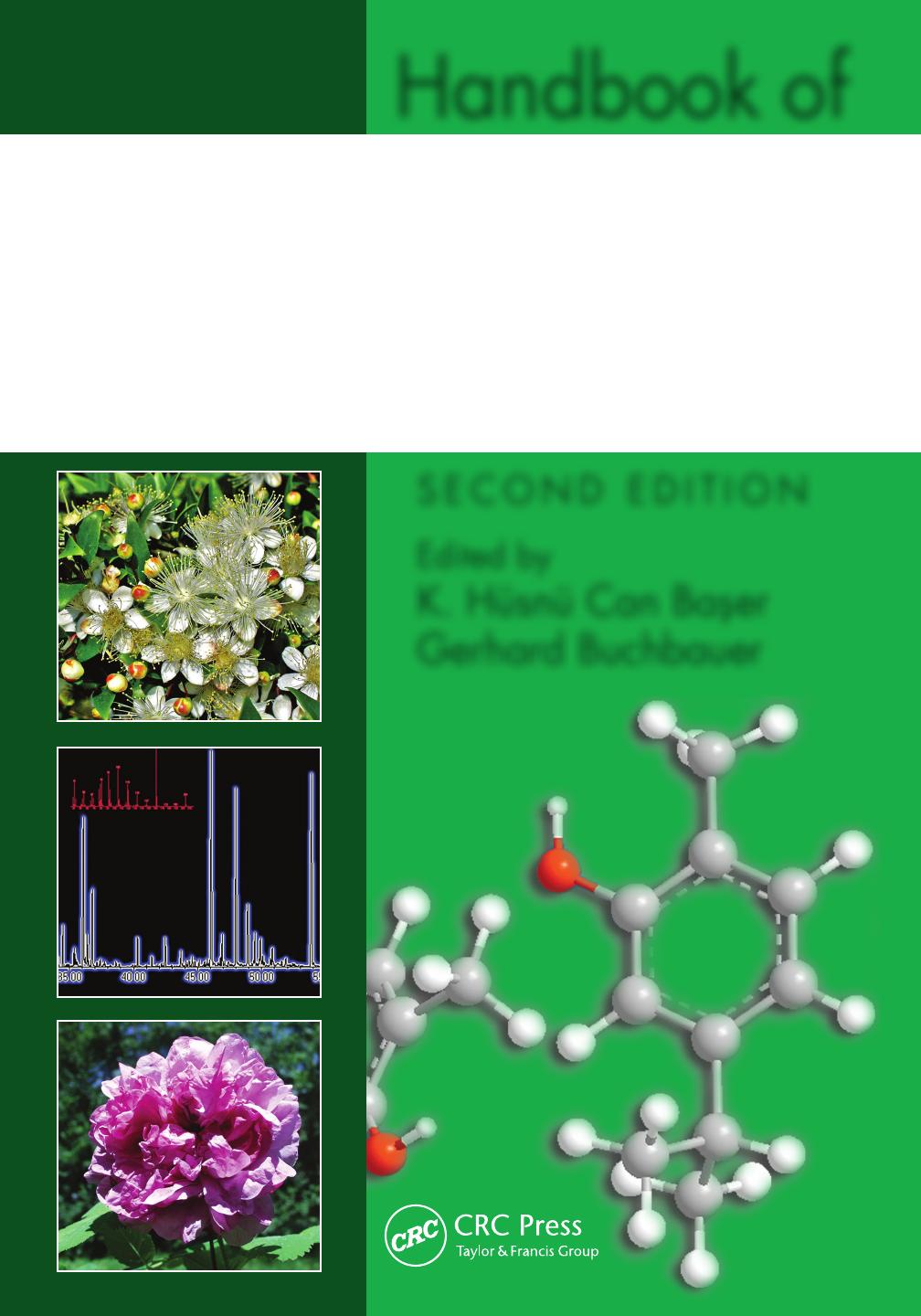
6000 Broken Sound Parkway, NW
Suite 300, Boca Raton, FL 33487
711 Third Avenue
New York, NY 10017
2 Park Square, Milton Park
Abingdon, Oxon OX14 4RN, UK
an informa business
www.crcpress.com
ISBN: 978-1-4665-9046-5
9 781466 590465
90000
K20466
www.crcpress.com
Handbook of ESSENTIAL OILS
Science, Technology, and Applications
Handbook of
ESSENTIAL OILS
SECOND EDITION
CHEMISTRY
Edited by
K. Hüsnü Can Bas
‚er
Gerhard Buchbauer
ESSENTIAL
OILS
Handbook of
Science, Technology,
and Applications
Bas
‚er
•
Buchbauer
SECOND EDITION
SECOND EDITION
The second edition of Handbook of Essential Oils: Science, Technology, and
Applications provides a much-needed compilation of information related to the de-
velopment, use, and marketing of essential oils. It focuses particularly on the chemis-
try, pharmacology, and biological activities of essential oils, with contributions from a
worldwide group of expert authorities on their historical, biological, regulatory, and
biotransformation aspects.
Features in the Second Edition
• New chapters on the natural variability of components that often occurs in essential
oils and on the natural processes that cause adulteration of essential oils
• Revisions, updates, and expansions of previous chapters
• Splits a previous chapter on biological activities of essential oils into two separate
chapters on biological activities and antioxidative properties
The book covers a wide range of areas applicable to essential oils research, including
sources, production, analysis, storage, transport, chemistry, aromatherapy, pharmacolo-
gy, toxicology, metabolism, technology, biotransformation, application, utilization, and
trade. It includes discussions of biological activity testing, results of antimicrobial and
antioxidant tests, and penetration-enhancing activities useful in drug delivery.
A continuation of its preceding edition that features enhanced and expanded informa-
tion, the second edition of Handbook of Essential Oils provides you with a broad
and authoritative knowledge base in the study and use of essential oils.