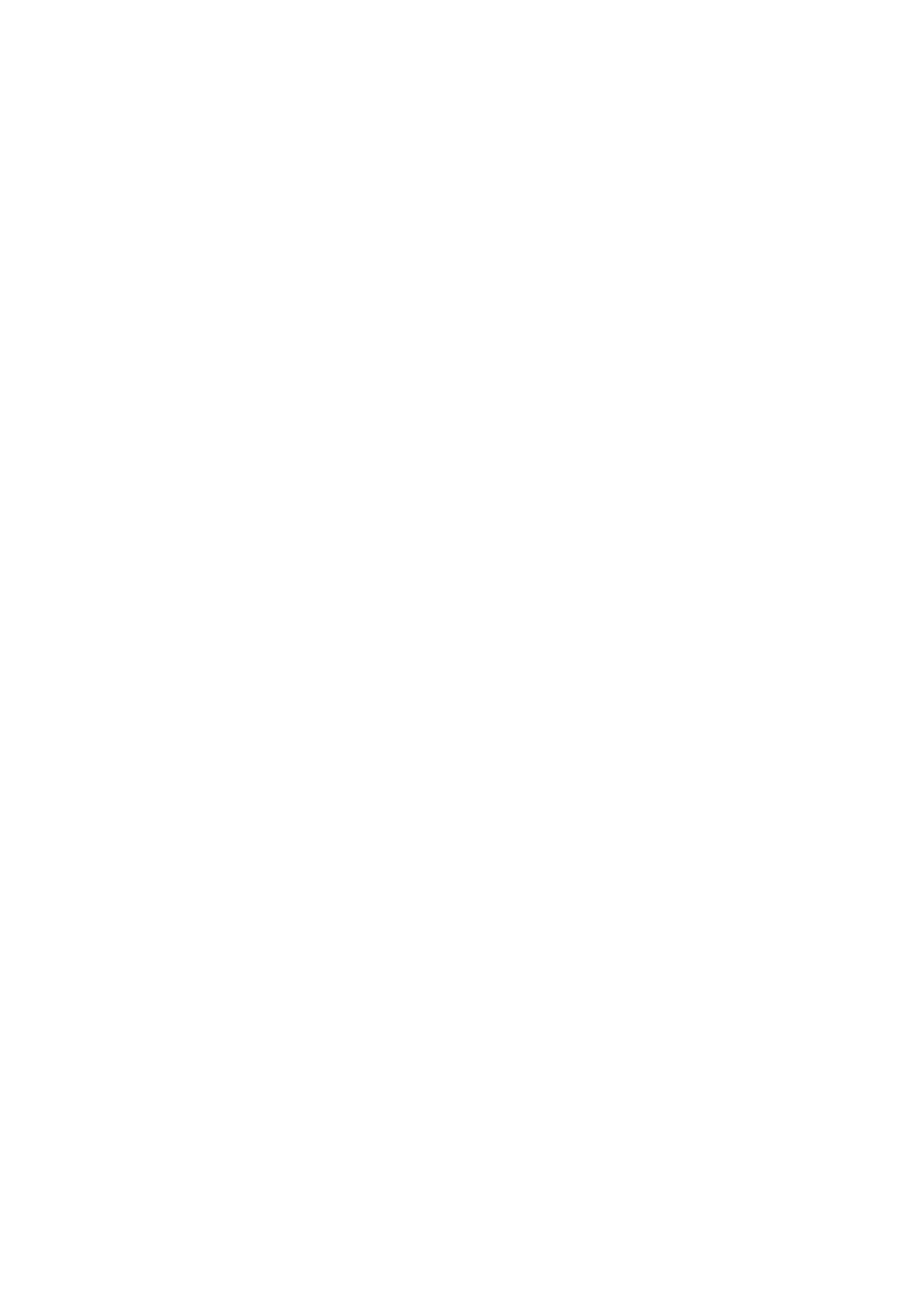
2
Chevaleyre. L’énergie consacrée par Claire pour le travail de paillasse de ma thèse m’a été
d’une grande aide. Merci Claire ! Grâce à toi l’élevage et les viscères du porc n’ont plus de
secret pour moi ! Tu as su me donner la passion du pot de labo, base essentielle du bon
fonctionnement d’une équipe. C’est pour cela que je dis vive Clairette !
Merci à Sandrine Melo aussi pour le travail de paillasse et le travail en élevage. Sa
disponibilité et sa bonne humeur m’ont été d’une grande aide dans la période chargée d’une
deuxième année.
Enfin un thésard dans un laboratoire, c’est comme un petit fusible, il en faut plusieurs sinon
ca pète ! Je tiens donc à exprimer ma gratitude envers Galliano Zanello (avec deux « l » svp !)
avec qui j’ai passé beaucoup de temps durant ces trois années. Pas trop au travail pour éviter
les accidents au scalpel, beaucoup à midi et en co-voiturage ! Mais surtout beaucoup en
dehors de l’INRA, merci Galli pour m’avoir amené au rugby, fait rire en VTT et parler dans
les bars !
La vie entre thésard masculin pourrait aussi lâcher sans la présence féminine. Une autre
thésarde a beaucoup compté dans mon intégration en Touraine, j’ai cité Marianne ! Merci
pour ta bonne humeur, tes soirées (chantantes) et ton écoute.
Parmi les autres jeunes de l’INRA, je souhaite remercier Manon (parce qu’elle est
Normande), Bertrand et sa petite taille, Denis et Thomas compagnons de squash, Astrid (vive
Preuilly !), Vincent DJ en devenir, Salim et son fameux tajine, Audrey, Nadia (vive
Grenoble !) et Fatemeh (ah ! Paris...).
Mes remerciements vont aux autres amis que j’ai pu rencontrer à Tours, les potes du rugby.
Aux amis de master de Grenoble, à ptit flo et enfin à mon ami d’enfance Arnaud.
Merci, pour leur collaboration scientifique, à Isabelle Lantier avec qui rime PCR et
microcapillaire. De même à Patrick Dabert pour son accueil à Rennes et ses connaissances en
SSCP. Merci aux unités expérimentales, Eric, Romuald, Frédéric, Eric, Gilles, Albert, Jean-
Philippe. Merci aux autres personnes de IASP avec qui j’ai pu partager des moments simples
au café, en congrès.
La rencontre de toutes ces personnes m’a aussi conduit à la rencontre essentielle de ma thèse.
La rencontre avec Lucie m’a donné l’énergie pour remonter des abysses et surfer sur les
vagues dangereuses qui font face au parcours d’un thésard. Merci mon chaton !!