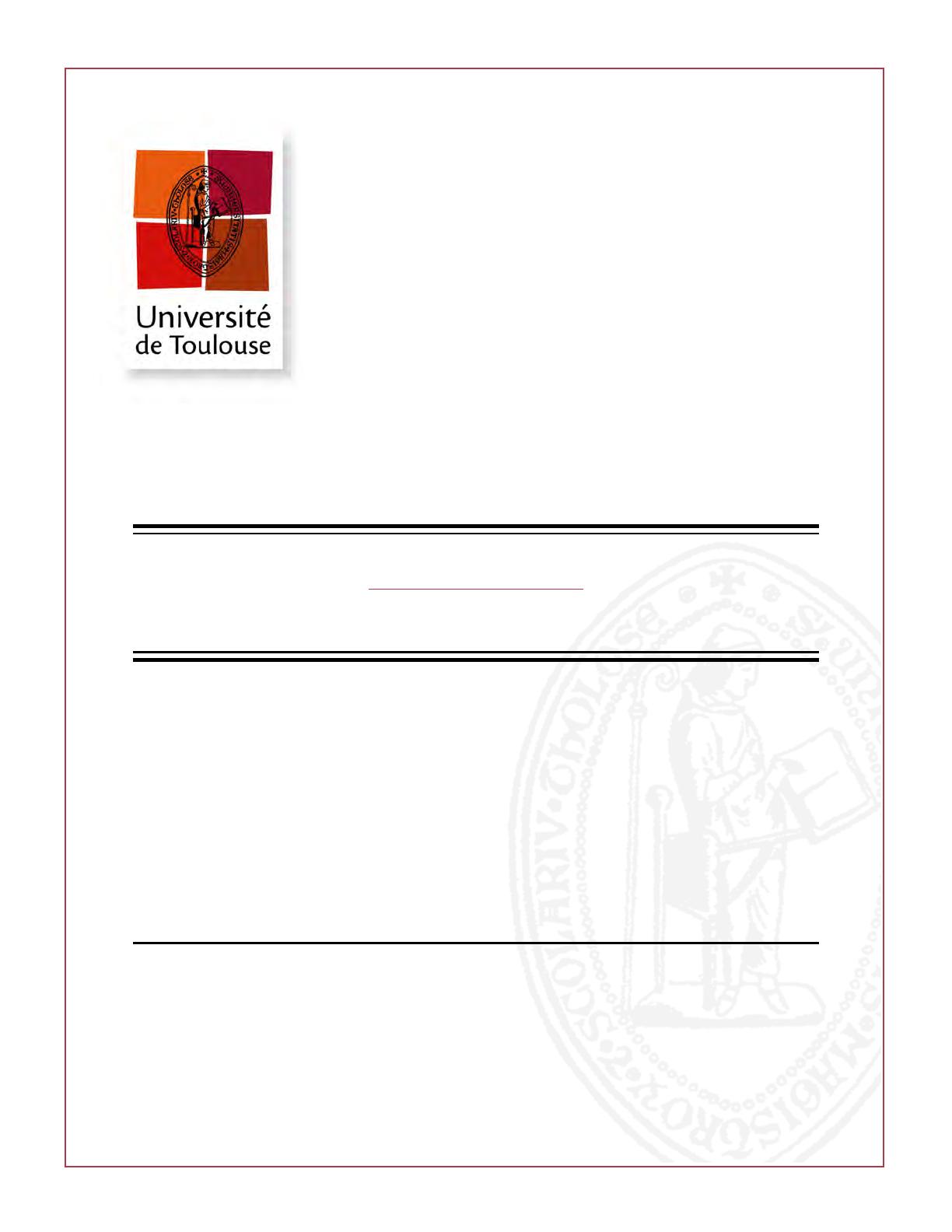
TH `
ESE
TH `
ESE
En vue de l’obtention du
DOCTORAT DE L’UNIVERSIT´
E DE TOULOUSE
D´
elivr´
e par : l’Universit´e Toulouse 3 Paul Sabatier (UT3 Paul Sabatier)
Pr´
esent´
ee et soutenue le 30/05/2016 par :
Minh Quan PHAM
Bio-pharmacological screening on liver-protective and
anti-hepatocarcinoma activities of Vietnam natural products
JURY
M. Etienne CHATELUT, Professeur, Universit´e Paul Sabatier, Toulouse Pr´esident du Jury
M. Lars Petter JORDHEIM, MCU-HDR, INSERM U1052 - CNRS 5286, Lyon Rapporteur
M. Georges MASSIOT, Directeur de Recherche, URCA, Reims Rapporteur
M. Bruno DAVID, Docteur es-Sciences, Institut de Recherche Pierre FABRE Examinateur
M. Quoc Long PHAM, Professeur, INPC-VAST, Vietnam Examinateur
M. Jean Edouard GAIRIN, Professeur, Universit´e Paul Sabatier, Toulouse Directeur de th`ese
´
Ecole doctorale et sp´
ecialit´
e :
BSB : Pharmacologie
Unit´
e de Recherche :
PHARMA DEV, IRD - Universit´e Paul Sabatier (UMR 152)
Directeur de Th`
ese :
Pr. Jean Edouard GAIRIN