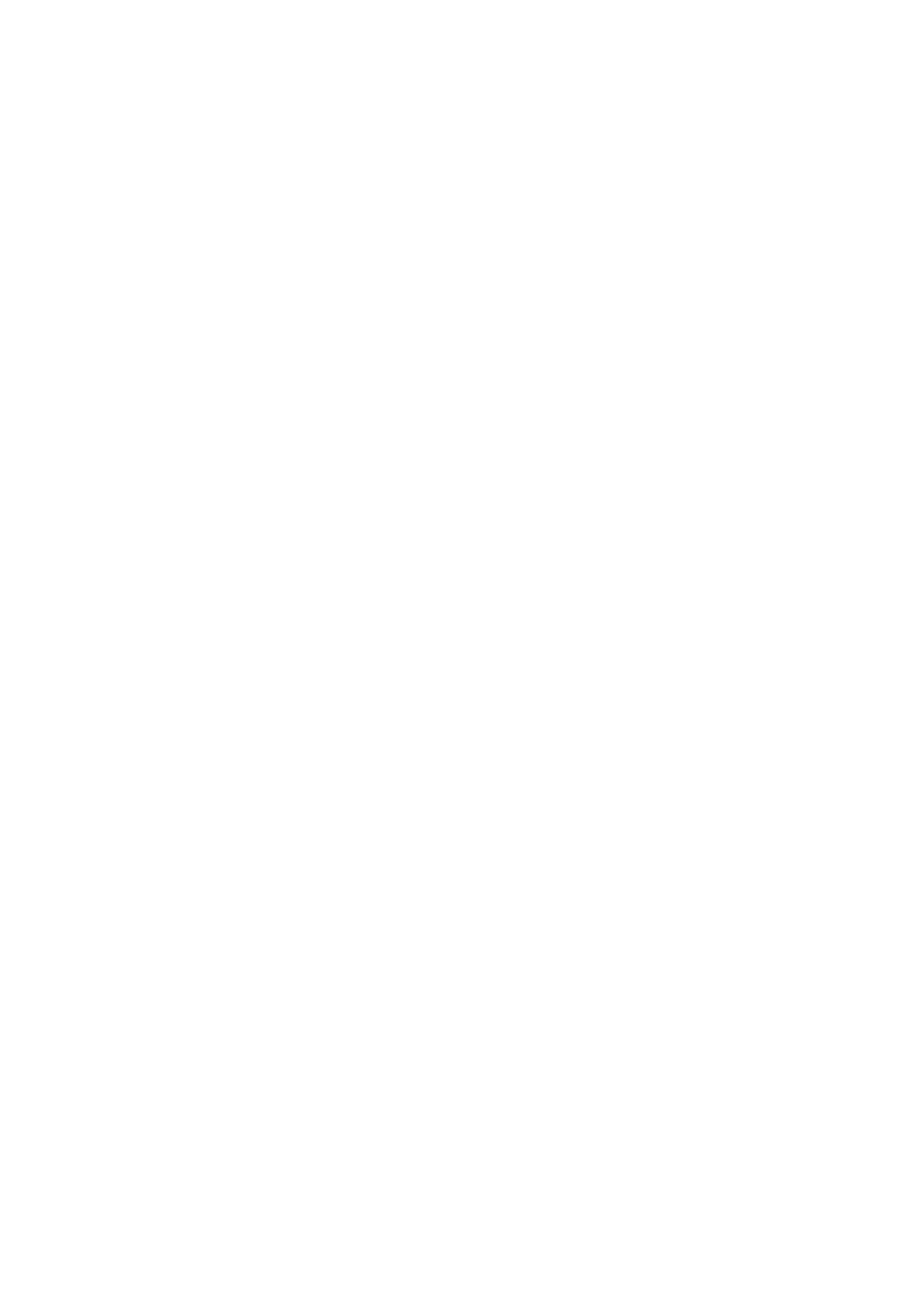
Acknowledgments
3
To Etienne, for his support, his restless willing to help, his not-being-afraid-of-speaking-English,
his peacefulness, and for making me feel welcome in France, I would like to thank him.
To Laetitia, Leticia, tia, mitia, myty, la-cruz, lacro-iX. You were my biggest support during these 3
years in the lab, I am not sure where I would be without you. You were my technical and emotional support,
my always-reassuring Plan B – SOS!, the person whose motivation inspired all the people in the team, and
who best represented the ‘crazy team’. The way you got through all the issues in your life is inspirational, and
I have no words to thank you. Thank you my Tia; I will get you back the favor one day (If you know what I
meeeaaan…Boulangerie!)
To the combo latino: Estefania, Claudia y Ana. Por hacerme dar cuenta que los latinos somos
mas chidos que todos los demás. Por su calidez, sus sonrisas, su comida, sus risas, las clases de español
latino, las risas y las risas. Qué lindo haberlas encontrado, las quiero mucho.
To Benedicte TicTic (or ‘Dic Dic’), thanks you for your jokes, you willing to help, your
motivational energy, your rapid style tips and reminding me that I am much much funnier and intelligent
when I talk Spanish. If only you’d know how funny I am in Spanish…
To Benedicte BenBen, for her willing to help, her scientific curiosity, for reminding us all how old
we are, for the restaurant tips, quick medical reviews and the superb massages, I am very grateful. (More for
the massages than anything else ;-)) I’m pretty sure you will be an excellent doctor and teacher one day
BenBen; I am proud of you and I am very glad I could participate (a little bit) to your formation as a
researcher.
To Ivo, thanks for your craziness, your craziness, your craziness and your craziness. You are the
proof that magical thoughts can still make their way into science. Your dedication and eagerness was
inspirational, and extremely helpful!
To Sophie Chauvet, la vampira, for her kindness, her generosity, her humbleness, her humor, her
tranquility. I felt so welcomed by you, and I am very glad I met you Sophie. You are more than welcome in
Colombia (or wherever I will be) when you feel like going for an adventure.
To Priyanka, thank you for your humility Priyankita, your humor, your smile, your vibrant energy
and your tranquillity. Your dedication and commitment with work is admirable, and I really hope you’ll fulfill
all your dreams (and I am pretty sure you will).
To Claire-la-petite, petite-Claire, for welcoming me in the lab, for your open-mindedness, your
willing to help; you were the little voice in my head that render me a bit anxious during my whole thesis.
To Estelle and Helene, for being the funniest, nicest, most helpful people in the CRC. Thank you
for teaching me such a great amount of stuff, for reminding me how much I like Flow Cytometry, for
making the long cell-sortings into funny moments. Thank you for being so welcoming, I hope we can work
again in the future.
To Nathalie Jupiter, pour ton aide, ta gentillesse, ton excellente cuisine, tes éclats de rire, ta bonne
humeur et ton envie irrépressible d’aider les gens, j’en suis extrêmement reconnaissant. Je suis content de
t’avoir croise sur ma route, tu rends les choses beaucoup plus faciles au laboratoire.
And last but not least, to Sarah for your loud laugh and the 100000 hours you dedicated to correct
my English! To Anne for her crazy-sympathetic-funny-open-minded personality. To Yann for his kindness
and all his help. To Tessa for her super-crazy craziness, her racist jokes, accusing me instead of Amelie for
stealing her Daim during 2 years!, and for the extra-kg I gained because of all the chocolates she gave me. To
Lucie for her kindness, her disposition for helping us, her violent massages and all the funny (way too
intimate) discussions. To Claire La Grande for all her help, the interesting discussions, her inspirational
dedication and commitment with work, her borderline ‘TOC’, for teaching us how important it is to wash
our hands and to take a prophylactic morning coffee to avoid the ‘morning mood’. To Myriam for
reminding me of my sister, and for her eagerness to discuss and learn. Enfin, to all other people in the lab,
Nicolas, Benoit, Hanane, Marion, Remi, Samantha, Kris, Helene, Pauline, Jerome, Shambu,