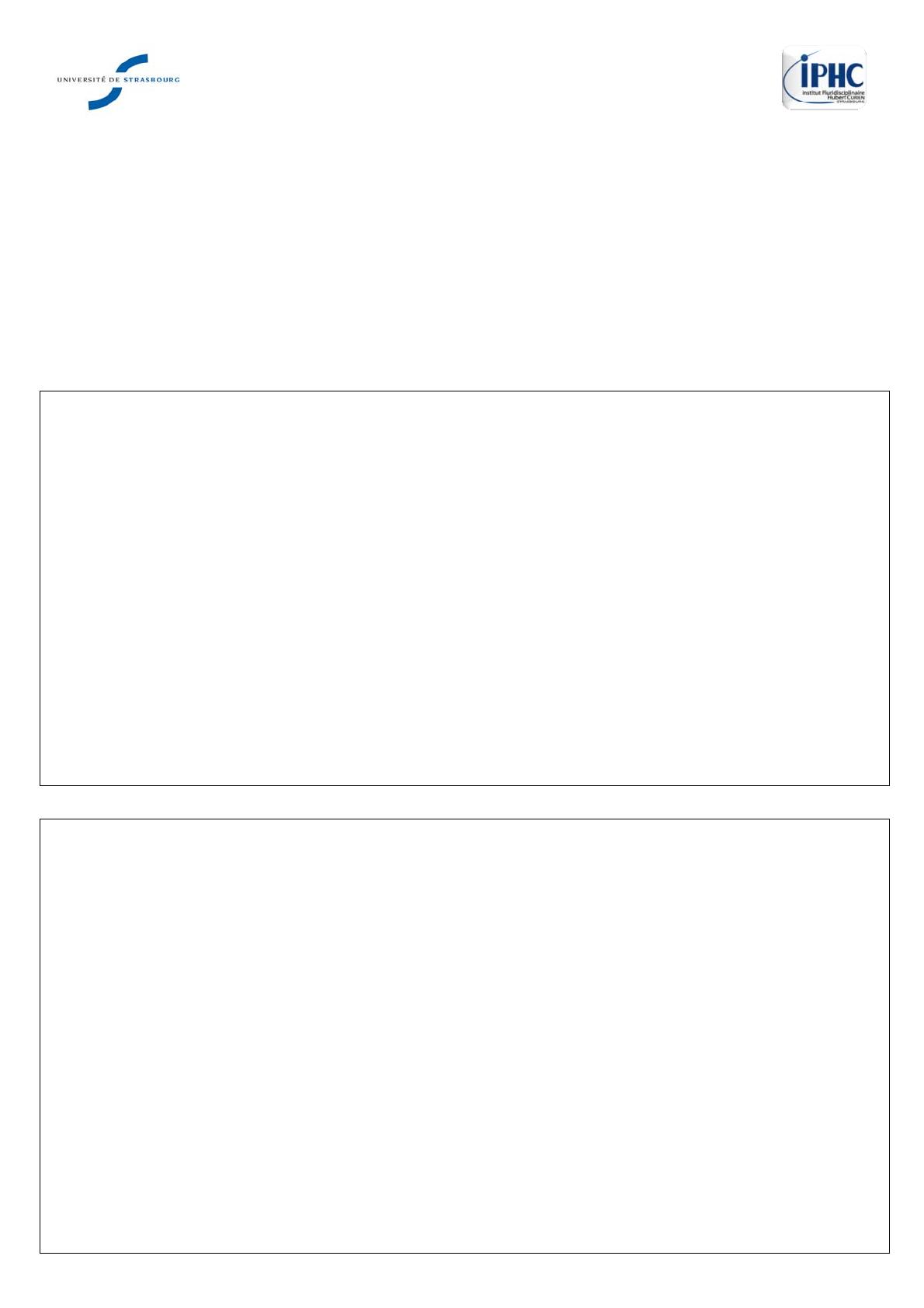
Alexia Arpel
DEVELOPPEMENT
PRECLINIQUE DE PEPTIDES
THERAPEUTIQUES
TRANSMEMBRANAIRES
APPLIQUES AU TRAITEMENT DU
CANCER DU SEIN
Résumé
Résumé en anglais
Le domaine transmembranaire des récepteurs membranaires est aujourd’hui considéré comme essentiel
dans l’activation et la régulation des voies de signalisation sous-jacentes. Ceci est tout particulièrement le
cas pour neuropiline-1 et -2 (NRP1/2), et ErbB2, trois récepteurs impliqués dans la croissance tumorale.
Notre laboratoire a initialement démontré qu’un peptide ciblant le domaine transmembrane du récepteur
NRP1, bloque l’oligomérisation de ce récepteur et provoque ainsi l’inhibition de la prolifération/migration des
cellules tumorales et l’angiogenèse in vivo. L’objectif principal de ce travail de thèse était d’élargir cette
stratégie aux récepteurs membranaires NRP2 et ErbB2, et ce, dans le contexte du cancer du sein. Mes
travaux montrent que ces peptides inhibent la pousse tumorale et les métastases associées dans différents
modèles de cancer du sein. Les effets anti-tumoraux peuvent s’expliquer par les propriétés anti-
angiogéniques et anti-prolifératives des peptides démontrées in vitro et in vivo. J’ai également disséqué le
mécanisme d’action du peptide ErbB2 et montré que le peptide inhibiteur de NRP2 induit des effets
secondaires rédhibitoires (promotion des métastases osseuses). Dans l’ensemble, mes recherches valident
le potentiel thérapeutique de cette stratégie peptidique et renforce l’idée d’un développement clinique de ces
composés. D’une terre inconnue à une terre d’espoir, le cœur de la membrane est incontestablement une
nouvelle source d’inspiration pour le développement des médicaments de demain.
Cancer du sein, Peptides transmembranaires, Neuropiline-1, Neuropiline-2, HER2, Métastases, Etude
préclinique, Imagerie
The role of transmembrane domains (TMD) in membrane receptor activation and regulation is nowadays
appearing as a key step of cell signaling. This has been indeed evaluated for neuropilin-1 and -2 (NRP1/2)
and ErbB2 receptors, three membrane receptors whose signaling has clearly been implicated in
tumorigenesis. Our team had demonstrated that a synthetic peptide blocking the transmembrane domain of
NRP1 blocked NRP1-dependent signaling leading to the inhibition of glioma cell proliferation/migration and
tumor associated angiogenesis in vivo. The major goal of this thesis project was to extend this novel strategy
to NRP2 and ErbB2 in the breast cancer context. Thus, I was able to demonstrate for the first time that the
use of peptides, inhibiting the TMD of these receptors, was able to inhibit tumor growth and related
metastases in vivo, in three different breast cancer mouse models that I have developed in the laboratory.
These results were supported by in vitro experiments demonstrating anti-proliferative and anti-angiogenic
properties of these peptides. Besides, I was able to dissect the mechanism of action of the peptide targeting
ErbB2 receptor in vitro and in vivo, and I provided data excluding NRP2 as a target because of an
unexpected promotion of bone metastasis. Altogether, my data offer convincing evidences to further develop
MTP-ErbB2 and MTP-NRP1 peptides as novel therapeutic compounds for patients suffering metastatic
cancers. From terra incognita to the exploration of a world of hope, the heart of the membrane is becoming a
new promising estate for drug design.
Breast cancer, Transmembrane peptides, Neuropilin-1, Neuropilin-2, HER2, Metastasis, Preclinical studies,
Imaging